Gate-tunable heavy fermions in a moiré Kondo lattice
Nature
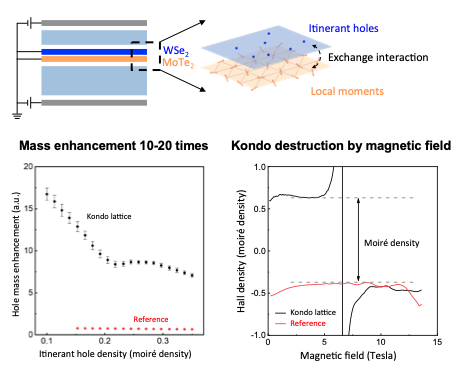
PARADIM Highlight #74
Common approaches to realizing strongly correlated quantum materials rely on intermetallic compounds that involve heavy elements like lanthanides. The use of naturally occurring elements limits the found material’s tuneability. Further, each atom consists of several dozens of electrons creating a very complex electronic structure, which make them hard to describe and predict by theory.
Here, members of PARADIM’s In-House Research Team demonstrate a model system created by stacking a pair of monolayer semiconductors, providing a simpler way to study confounding quantum behavior, from heavy fermions to exotic quantum phase transitions.
The team uses MoTe2/WSe2 moiré bilayers, in which the MoTe2 layer is tuned to a Mott insulating state, supporting a triangular moiré lattice of local moments, and the WSe2 layer is doped with itinerant conduction carriers. They observe heavy fermions with a large Fermi surface below the Kondo temperature and that the heavy fermions be destructed by an external magnetic field. The Kondo temperature can be tuned widely and continuously via an applied electric voltage. The study opens the possibility of in situ access to the phase diagram of the Kondo lattice with exotic quantum criticalities in a single device based on semiconductor moiré materials.
What has been achieved:
The Kondo lattice—a matrix of local magnetic moments coupled through spin-exchange interactions to itinerant conduction electrons—is a prototype of strongly correlated quantum matter. Usually, Kondo lattices are realized in intermetallic compounds containing lanthanide or actinide. The complex electronic structure and limited tunability of both the electron density and exchange interactions in these bulk materials pose considerable challenges to studying Kondo lattice physics. Here PARADIM researchers report the realization of a synthetic Kondo lattice in AB-stacked MoTe2/WSe2 moiré bilayers, in which the MoTe2 layer is tuned to a Mott insulating state, supporting a triangular moiré lattice of local moments, and the WSe2 layer is doped with itinerant conduction carriers. They observe heavy fermions with a large Fermi surface below the Kondo temperature. Further, they observe the destruction of the heavy fermions by an external magnetic field with an abrupt decrease in the Fermi surface size and quasi-particle mass. The work also demonstrates widely and continuously gate-tunable Kondo temperatures through either the itinerant carrier density or the Kondo interaction. The study opens the possibility of in situ access to the phase diagram of the Kondo lattice with exotic quantum criticalities in a single device based on semiconductor moiré materials.
Importance of the Achievement:
Realization of a synthetic Kondo lattice model presents a unique opportunity to explore the origins of novel quantum phase transitions and phenomena, including non-Fermi liquid behavior, heavy-fermion superconductivity, Kondo insulator, topological Kondo insulator, quantum criticality of magnetic instability, and more. With the gate tunability, the different exotic quantum phases have chance to be realized in a single device. The quantum critical point or phase can be studied in details by fine-tuning the gate voltages.
Unique Feature(s) of the MIP that Enabled this Achievement:
Making good devices relies on a complete fabrication platform with cut-edge instruments. PARADIM is such a platform with all essential tools needed for device fabrication.
The work was conceived and performed by members of PARADIM’s In-House Research Team.
Full reference:
W. Zhao, B. Shen, Z. Tao, Z. Han, K. Kang, K. Watanabe, T. Taniguchi, K.F. Mak, and J. Shan, "Gate-Tunable Heavy Fermions in a Moiré Kondo Lattice," Nature 616, 61–65 (2023). DOI: 10.1038/s41586-023-05800-7
Acknowledgments:
We thank L. Fu, D. Guerci, A. Millis, A. Georges, A. Rubio, S. Todadri, A. Kumar, A. Potter, D. Chowdhury and Y. Zhang for discussions. This work was supported by the Air Force Office of Scientific Research under award number FA9550-19-1-0390 (transport measurements), the National Science Foundation (Platform for the Accelerated Realization, Analysis, and Discovery of Interface Materials) under cooperative agreement nos. DMR-2039380 (sample and device fabrication) and DMR-2004451 (optical measurements) and the US Department of Energy, Office of Science, Basic Energy Sciences, under award number DE-SC0019481 (analysis). This work is also funded in part by the Gordon and Betty Moore Foundation. The growth of the hBN crystals was supported by the Elemental Strategy Initiative of MEXT, Japan, and CREST (JPMJCR15F3), JST. We used the Cornell Center for Materials Research Shared Facilities supported through the NSF MRSEC programme (DMR-1719875) and of the Cornell NanoScale Facility, an NNCI member supported by NSF grant NNCI-2025233. We also acknowledge support from the David and Lucille Packard Fellowship (K.F.M.) and the Kavli Postdoctoral Fellowship (W.Z.).